di Davide Sivolella
Two-hour drive north of Los Angeles at the southern edge of the Antelope Valley portion of the Mojave Desert, Palmdale and its neighbourhoods play host to some of the most advanced past and present undertakings of the US aerospace industry. Driving around the local regional airport you will easily spot hangars belonging to distinguished names such as Boeing, Lockheed Martin, Northrop Grumman, and NASA.
Today, I am at the Building 703 of the NASA Neil Armstrong Flight Research Center at the southern end of the local airport and I am about to fly on a one-of-a-kind machine that marries aeronautical engineering with astronomy. It is the Stratospheric Observatory For Infrared Astronomy (SOFIA), a Boeing B747 aircraft whose aft fuselage has been heavily modified to carry a 2.7-m Cassegrain reflector telescope designed to detect infrared light. Simply put, SOFIA is an airborne observatory. Its origins can be traced to astronomers Gerard P. Kuiper and Frederic F. Forbes who in 1965 flew a Convair 990 jet airliner owned by NASA and modified to house instruments looking at the sky through the fuselage passenger windows. They recognized that at the typical altitude flown by a jet airliner, observations in the infrared light would be enabled as no longer obstructed by the water vapour in the atmosphere below. With such an apparatus, the two astronomers were able to measure the near-infrared spectrum emitted by the Venusian cloud top and discover that the atmosphere of Earth’s twin is essentially dry. It was a significant moment for the scientific community as it established evidence that to conduct meaningful infrared astronomy you need not necessarily to rely on observatories at orbital altitude. Because the NASA Convair 990, also known as Galileo I, was also demanded for other research activities, staff at the NASA Ames Research Center modified a small Learjet business jet to carry a 12-inch diameter open-port telescope. Now, not only the astronomers had an aircraft dedicated exclusively to infrared astronomy but also a telescope that could observe through a cut out in the aircraft fuselage and thus enable detection of longer far-infrared wavelengths. On Galileo I, because the telescope used by Kuiper and Forbes was constrained to look through a glass window, detection of longer far-infrared wavelengths was precluded. The successful observations carried out with Galileo I and the Learjet reinforced the notion that airborne astronomy was worth undertaking. To enable a deeper probing into the infrared universe, the early 1970s saw the entry into service of the Kuiper Airborne Observatory (KAO). KAO was a civilian variant of the Lockheed C-141 Starlifter, a four-engine military jet transport, that featured a 36-inch telescope installed into a cavity that penetrated the left fuselage just forward of the wing root. Three times the diameter of the Learjet telescope, the KAO telescope gathered nine times the amount of light, enabling longer observations with dramatically more sophisticated and sensitive apparatus. Among the many discoveries logged by KAO we can mention the detection of water in the thick atmosphere of Jupiter; the determination that Uranus has not an internal heat source; the investigation of objects called “Bok Globules (named after Harvard astronomer Bart Jan Bok) proving that rather than being just boring black patches in the night sky they are regions of stellar formation; and the analysis of supernovae such as the famed SN1987A, a supernova event that took place in 1987 in the Large Magellanic Cloud, a dwarf galaxy satellite of the Milky Way.
KAO’s 20 years of operations wet even more the appetite of the infrared astronomy community for airborne astronomy. During the KAO program, NASA had collaborated extensively with German astronomers. Thanks to such a fruitful collaboration, NASA and DLR, the German Space Agency, decided to join forces in developing KAO’s successor, giving birth to the SOFIA program. General speaking SOFIA, the “Stratospheric Observatory for Infrared Astronomy” is a joint project of the Deutsches Zentrum für Luft- und Raumfahrt e.V. (DLR) and the National Aeronautics and Space Administration (NASA). It is funded on behalf of DLR by the Federal Ministry for Economic Affairs and Energy based on legislation by the German Parliament, the State of Baden-Württemberg and the University of Stuttgart. Scientific operation for Germany is coordinated by the German SOFIA-Institute (DSI) of the University of Stuttgart, and in the USA by the Universities Space Research Association (USRA).
As far as the aircraft was concerned, in 1997 they acquired a retired Boeing 747SP. Based on the airframe of the B747-200 (a so-called “classic” version of the Jumbo Jet), the B747SP was designed in the early 1970s to fill the ultra-long-range market niche, such as nonstop flights between city pairs like Sydney-Los Angeles, Johannesburg-London, and New York-Tokyo. To enable such performance, the B747-200 fuselage was shorted by approximately 15 meters, the vertical and horizontal stabilizers were lengthened by 1.5 meters, and the fuselage tail section was reinforced and re-contoured. The type, however, did not appeal airlines as much as Boeing hoped, and in 8 years of production, only 46 aircraft were built. That is a shame, as it is a truly elegant aircraft, pleasant to the eye and admired by aviation geeks all over the world.
As I enter the cavernous hangar space of Building 703, I have my first up-close view of this unique flying machine. As she is being towed and parked into an adjacent stand, her white livery interspersed with NASA, DLR, USRA and SOFIA decals and marked with a blue cheat line along the passenger window row, shines in the already intense morning sun under a cloudless sky. Under the terms of this NASA-DLR joint venture, the American space agency took responsibility for the airframe structural modifications, while the German space agency took charge of the design and commission of the telescope. The DLR also contracted the University of Stuttgart to maintain and further develop the telescope throughout its projected 20-year lifespan, which also includes special missions. To do so, the Deutsches SOFIA Institut (DSI) was established and it is thanks to the DSI that today I can experience what it means flying and operating SOFIA. I am being escorted by Dr. Dörte Mehlert, an astronomer responsible for DSI’s Education and Public Outreach activities, and Nadine Fischer, a DSI software engineer permanently based at Palmdale.
We begin with a walk around the aircraft. My attention is immediately caught by the bulging behind the wing. This is where a 5.5 x 4.1 m section of left hand fuselage has been removed to fashion the cavity that houses the telescope. Essentially, one quarter of the fuselage cross-section has been removed to position the telescope inside the fuselage. A large door covers the telescope cavity on the ground. During flight, like an eyelid, the door slides over and around the fuselage towards the right side, exposing the telescope to the atmosphere. To recover the structural strength and rigidity that such an invasive cut entails, the structure around the cutout is reinforced with several layers of overlapping plates and straps. Aerodynamic fairings encircle the cutout to restore a smooth fuselage profile at this location.


Once on board, Dörte and Nadine guide me through the extensive modifications undertaken to transform a passenger airliner into an airborne observatory. Just aft of the wing, a hefty bulkhead has been erected spanning the full diameter of the fuselage. The bulkhead is the border between the pressurised cabin, where the crew operates during flight, and the telescope cavity. In fact, the crew would not survive if exposed to the atmosphere when the cavity door is open. Hence, in flight the crew cannot access the telescope cavity. The bulkhead is also the pivot point of the whole 17-ton telescope assembly, which has been designed as a dumbbell. The end within the aft fuselage cavity houses the telescope optics; the extremity inside the crew cabin accommodates the supporting structure to mount the science instrument selected for the flight. The two end points are connected by a stiff so-called Nasmyth tube, made of carbon fibers, designed to minimize the deformation of the dumbbell due to gravity. At the intersection between the bulkhead and the Nasmyth tube there is a 1.2-m diameter cast iron bearing floating on a 50-micron thick film of oil supplied by a reservoir housed in the forward cargo hold. This hydrostatic bearing completely isolates the telescope assembly from the aircraft in case of turbulence.


It is easy to appreciate the challenges involved in stabilizing a flying telescope despite the many disturbances produced by the aircraft. Therefore, Nadine spends some time in showing me the so-called Vibration Isolation System, or VIS. It consists of 24 air springs and three dampers arranged along two blue-painted rings. The air springs are pressurized with dry air from pressure bottles located in the aft compartment on the upper deck. Together they isolate the telescope from high-frequency vibrations caused by the aircraft’s engines and aero-elastic deformations of the wings and control surfaces. During take-off and landing it secures the telescope by pressing it against hard stops. A large 2.4-m diameter spur gear driven by four electric brushless motors are the main components of the coarse drive assembly that rotates the telescope between 20° to 60° in elevation from the horizon. A finer control of the telescope orientation is provided by the electromagnetic motors of the so-called fine drive assembly acting on all three axis around the spherical bearing. With it, ±2.7° adjustments in elevation, cross-elevation and line of sight can be performed. In addition, they also eliminate disturbances not completely arrested by the VIS as well as those caused by friction in the bearing, seals and cables. During changes in the telescope elevation, the fine and course drive are coupled. At any other moment, they operate independently of each other and of VIS.
For today’s flight, the observations will be carried out with the Far Infrared Field-Imaging Line Spectrometer, or FIFI-LS. This instrument was developed in Germany and commissioned by DSI in 2014. It is now maintained and operated by the SOFIA Science Mission Operation Center. The instrument is made of two independent grating spectrometers and associated detector, each consisting of 400 pixels. One spectrometer operates at short wavelengths, between 50 and 125 micron; the other covers the range from 105 to 200 micron. As Dörte explains, FIFI-LS is a unique instrument as it designed for returning a 2D infrared image of the target as well as its infrared spectrum. The collected data is organized in a sort of 3D matrix, called the cube, which will then be analysed by the principle investigator(s). The instrument is cryogenically cooled with liquid helium and liquid nitrogen. As they gradually boil off in flight, the instrument must be refilled in the morning before a new mission. While in the cabin, I observe how this is performed by two technicians by connecting pressurised bottles to the tanks inside the instrument. The first refill is for the nitrogen, followed by the helium. The whole operation is rather uneventful and unsophisticated.
Typically, every 2 to 4 weeks there is an instrument change. Currently SOFIA can support 6 different instruments. These are stored in the instrument lab under the care of lab supervisor Gregory Perryman. Each instrument is transported on a dedicated cart. Before installation on the aircraft, Gregory and his team perform a test run on a mockup that reproduces exactly the Nasmyth tube and the mechanical interface with the instrument. They want to be certain that the cart is not damaged and can hold the instrument for an exact installation on the supporting structure of the Nasmyth tube. As Gregory explains, it is much better to identify any cart misalignment and rectify it in the lab, rather than on the aircraft where it might cause serious damage to the telescope! The instrument is then transported to the aircraft with a scissor lift platform, rolled into the cabin through the left-hand entry door just forward of the wing and then wheeled to the bulkhead. Mechanical, power and data connections are then made and the instrument is ready for flight. Typically, it takes one day to swap a science instrument. Every time the instrument is changed, the telescope assembly has to be rebalanced. In fact, regardless of the instrument, the telescope assembly’s center of gravity has to coincide with the center of rotation of the hydrostatic bearing. In this way, the torque required to rotate and keep the telescope pointed is minimized. Balancing is achieved by adding or removing steel plates fitted around the instrument interface flange. During flight, as the cryo coolants boil off, an electric motor slowly moves two sets of 80-kg weights, counteracting the reduction in instrument mass that the boil off entails. In this way, the center of mass of the assembly continues to coincide with the hydrostatic bearing’s center of rotation.







During flight, the crew works on dedicated stations. These are desks filled with monitors, computers, electronics and chairs not dissimilar to an ejection seat. Close to the bulkhead there is the station of the telescope operators, which is adjacent to that of the science instrument operators. In the middle of the cabin, there is the desk of the two mission directors. To the side, there are two tables to accommodate additional crew members, such as the principal investigators that have proposed the observations undertaken during the flight. A fourth workstation is dedicated to Education and Public Outreach activities to allow guests like myself to watch the progress of the flight and observations. At the very forward end, the former First Class cabin offers seats for extra guests or crew members. A number of large cabinets housing electronic equipment, cables and computers are interspersed throughout the cabin. I cannot help but notice the bulkiness of such equipment. This is not surprising, considering that such equipment was designed and built some two decades ago and that new and lighter modern electronic apparatus would need a lengthy certification before they can replace older equipment.
As my tour continues, a small maniple of people is preparing the aircraft for our evening flight. They are under the direction of crew chief Jerry Dobbins who oversees SOFIA’s daily maintenance. As Jerry explains, they spend some 6 hours every day carrying out internal and external detailed visual inspections of the whole airframe to make sure that it is safe and sound for the next flight. Compared to the less than 2 hours that typically an airline allocate for a quick inspection of a commercial passenger aircraft of equivalent size before next flight, SOFIA is indeed treated with the outmost care. She’s one of a kind, whose mission is to fulfil the hopes and objectives of the scientific community. In fact, throughout my visit I can easily recognise how everybody involved with SOFIA is highly committed to make her fly safely and promptly every night.
Because diagnostics of the previous flight had detected a noise emitted by a mechanical component common to the telescope assembly, supervisor and fellow countryman Marco Lentini is about to inspect the telescope cavity to assess the health of the component. I am given the opportunity to follow Marco in his inspection and this is a real treat as usually there is no need for post-flight assessment of the telescope. To enter, we have to don an overall, head cap, mouth mask and shoe gloves. We gain access through a small door cut into the cavity’s aft bulkhead. Once inside, there is enough room to walk comfortably around the telescope assembly and I have the opportunity of staring directly into the 2.7-m primary mirror. When on the ground, the telescope is locked at 40° of elevation. This is a default elevation maintained also for take-off and landing. As Marco explains, the primary mirror is housed into an open lattice work made of carbon fibre. This material not only offers a light rigid structure but also it does not deform easily. On the ground, the diurnal temperature of the Mojave Desert quickly warm up the telescope cavity and everything in it. In flight, when the door covering the cavity slides open, the telescope is subject to the punishing -40°C of the stratosphere. The carbon fibres’ low coefficient of thermal expansion (just a few percent of steel’s) prevents the telescope supporting structure from deforming, preserving the intended shape of the telescope assembly. The lattice structure is coated with a black paint, formulated to avoid an infrared glare that would deteriorate the quality of the light delivered to the scientific instrument. The mirror is coated with a thin layer of highly reflective aluminium, for a total mass of just one gram! The back side of the mirror is exposed and I can appreciate its honeycomb-like structure. In fact, the mirror was first cast as a monolithic body weighting some 3.5 tonnes. Then the backside was milled and etched into a ribbed structure with hexagonal pockets, lowering the weight of the mirror to “just” 900 kg. Finally, it was polished to the desired parabolic shape. From the primary mirror the light is focused on the secondary mirror, which then redirects the light towards the tertiary mirror that sits at the center of the primary mirror. From here, the light is channelled through the Nasmyth tube into the science instrument.

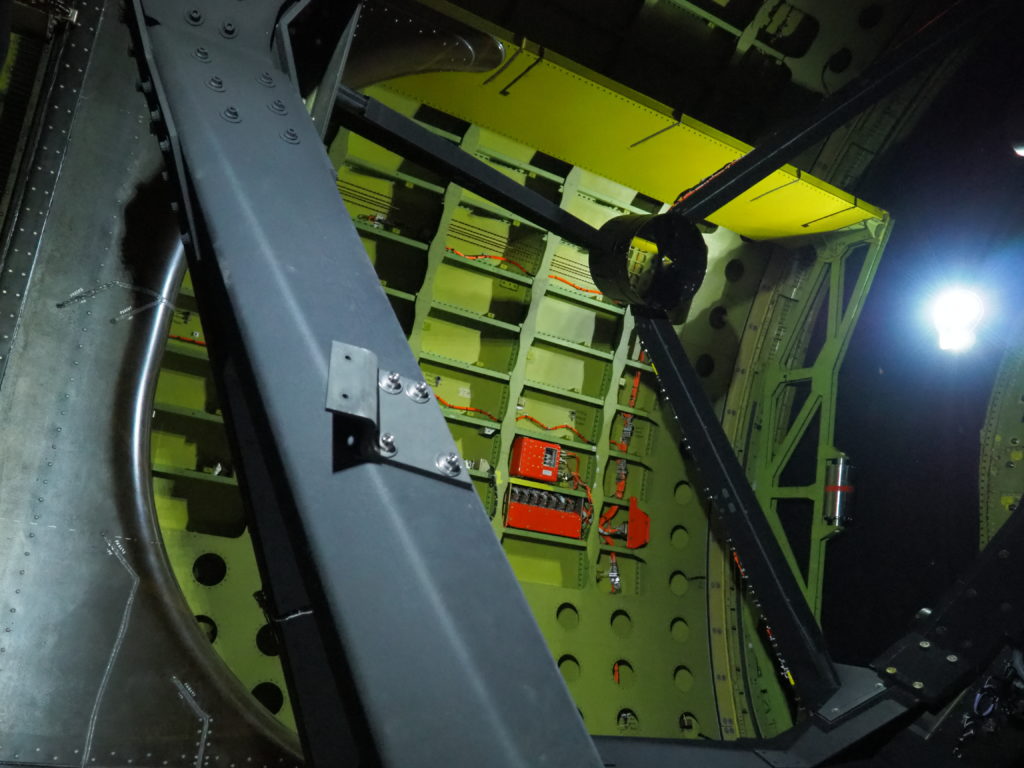
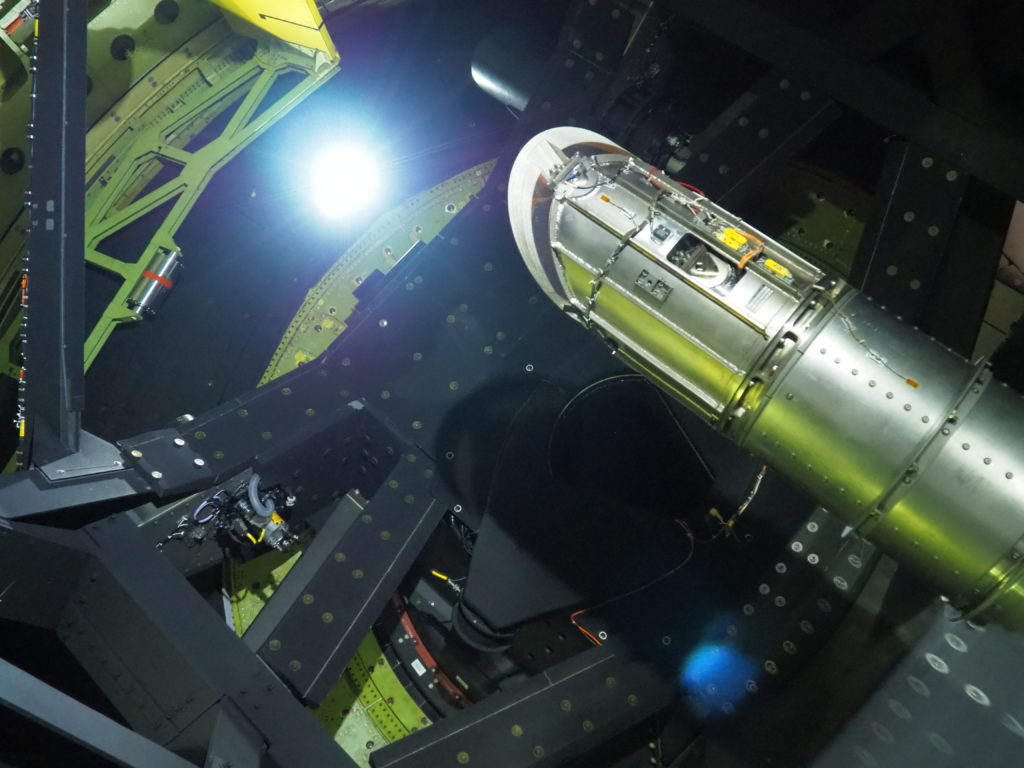
Before flight, I must attend an emergency egress training. This is not dissimilar to the typical safety briefing on a commercial airplane, but for SOFIA there are additional precautions to take. For instance, on board everybody has always to carry the so-called Emergency Personal Oxygen System, or EPOS. This is a cross body bag containing a small bottle of oxygen attached to a smoke hood. In case of fire, you don the smoke hood, so that your head is fully contained in it and you can continue to breath (do you remember when you were little, and your parents would tell you not to put on a plastic bag over your head?). In this way, you will not be overcome by the smoke and you can help extinguishing the fire or an injured crew member, if trained to do so. In case of rapid decompression, oxygen masks will automatically drop from the ceiling. If you prefer you can use your EPOS instead, and thus be free to move throughout the cabin should you need to do so (e.g.: to help a fellow crew member). The instructor also reminded us how to operate the exit doors and inflate the slides for rapid evacuation on the ground or in case of water landing. In this case, the slides double as rafts.
Some two hours before take-off, I attend the mission briefing where the objectives for the evening are laid out. Before that, the pilots along with safety officers have had their own briefing. We will start with the M82 galaxy to study extragalactic star formation processes. Then we will gather light from a region of intense star formation in our galaxy located in the Canis Major region. The aim is to trace how protostars can regulate the efficiency of star formation within a star cluster. Finally, we will study a pair of interacting spiral galaxies where the generation of energetic cosmic rays is poorly understood. As I am about to experience, the 16 crew I will be flying with are akin to an orchestra playing a complex symphony called flight plan. The flight plan is prepared months in advance and amended as the date of the flight nears. As astronomers file proposals for observations, a committee selects those with the highest scientific return. To each approved project, observatory time and priority are assigned. The individual projects are then grouped together by the instrument required for the observations, forming a so-called “target pool”. Each target pool is flown during a so-called “series” which, on average is 3 to 4 weeks long, although it can be shorter or longer. An automatic scheduling algorithm generates the individual flight plans for the series. It is an exercise in global optimisation in which 5 to 20 million schedules can be generated until when the best plan for a given flight is finalised.
The plan contains the sequence of targets to survey, based on constraints such as that SOFIA is to return to the point of departure, that the telescope can only look at the left, telescope range of movements, restricted airspaces, limitations on timing and location to initiate a given observation, time of raising and setting of the targets, and so on. Weeks before the beginning of the series, the flight plans are reviewed by the instrument scientists and tweaked where required. Once approved, they can only be slightly amended. Typically, this would occur the day before the flight or on the day itself, to account for up-to-date weather forecasts and changes to restricted airspaces. Each flight plan is divided into legs. A leg refers to the viewing of one target. Typically, the highest priority target of the flight is observed first, followed by targets of lesser importance (compared to the first). Typically the last leg is reserved for the so-called high-risk high-return targets, whose observation is known to be arduous but if successful can yield high scientific value.
One hour before take-off, the door is closed. If you are not onboard, you have missed your flight. I have been given permission to sit in the flight deck behind the pilots. That SOFIA is a vintage aircraft is evident as my seat is next to the flight engineer whose role is to look after all of the aircraft onboard systems, such as hydraulics, pneumatic, fuel, electric, and so on. He is also responsible for opening and closing the telescope cavity door when instructed to do so. The pilots are instead focused on flying the aircraft. Today’s the glamorous position of the flight engineer has been replaced by a computer, leaving only the pilots in the flight deck. Take off is at 20:03 and sure enough we depart sharply on time. The successful accomplishment of the flight plan relies on SOFIA being run as a Swiss watch. The departure must occur on time as each observation scheduled for the night relies on SOFIA being at an exact location in the sky at a precise time. A delay of even just a few minutes, can devastate the whole flight plan and yield angry astronomers. Windows of opportunity can be built into the flight plan to account for unforeseen events, but they necessarily entail either a shortening of observation time on a given leg or outright removal of an observation. For instance, I learn how a few weeks earlier a mechanical fault had prevented an on time departure. The flight plan conceded a half an hour and a two-hour window. The fault was cleared but not before the first window had elapsed. Hence, they had to wait the opening of the two-hour window before departing for a shortened mission. On the ground and before starting the engines, onboard power is supplied by the so-called Auxiliary Power Unit (APU), a small jet engine located on the aft fuselage beneath the fin. In flight, power is instead generated by the four engines. For the transfer from APU to engine-generated power, the telescope must be powered down. This prevents the power switch from damaging the delicate electronics of the telescope and instrument. The power switch is communicated to crew and pilot with the call “power transfer”.

After take-off we quickly clear the airspace around Los Angeles. I learn another clue that SOFIA is based on vintage technology by observing the pilots manually adjusting the thrust level of the individual engines, in order to maintain the required airspeed. Unlike modern airliners, SOFIA’s autopilot does not command the throttle. Surely, it makes it more fun to fly. As we reach 11,000 meters, the flight engineer receives the command to open the telescope door. He complies by just flicking a switch. There is no feeling at all of the door opening. The external fairings surrounding the telescope cavity are so-well designed that the airflow is barely disturbed. There is no buffeting or vibrations induced into the airframe. The empennage authority is not affected. It is like if the telescope cavity was not there. This is the remarkable result of hundreds of hours of wind tunnel testing and fluid-dynamic simulations. At this point I move into the main cabin. To help the science instrument remain cold and slow down the boil off of its cryogenic coolants, the insulation panels that would have typically lined the interior of the fuselage at this location are missing. Consequently, the cabin temperature is lower than that experienced on a commercial flight and if you add the natural tiredness that accompanies working at night and, in my case, also jet lag, the cabin does feel cold. For this reason, I was glad that Dörte had warned me beforehand to take with me some heavy clothing. From the take off to landing, the deafening screaming of the four Pratt & Whitney JT9D engines fills the cabin unabated, requiring that you wear an headset at all times that allows you to talk with anybody and listen to any conversation going on among the crew. Without headset, the most effective way of communicating would be by sign language!

Telescope operator Erik Sandberg shows me how this is done. First he inputs the coordinates of the target into the computer, which steers the telescope towards the generic area of the sky where the target is located. There are three guiding cameras: the Focal Plane Image (FPI+), the Wide Field Imager (WFI) and the Fine Field Imager (FFI). While FPI+ is mounted inside the cabin and at the same focus as the scientific instrument, the other two are mounted on the telescope supporting structure on the other side of the bulkhead, so that they experience the same environment as the telescope. Using the WFI camera, which has the largest field of view, Erik checks whether the telescope is pointing to the correct field in the sky by comparing a well-known star map with the image on the camera. Then with the FPI+ camera he looks for two guide stars. The FPI+ camera is ideally suited for this task as it has the smallest field of view but the highest sensitivity and is a very fast camera. If the selected stars belong to the star catalogue stored in the computer memory, Erik confirms to the computer that the two stars are the guide to keep the telescope pointed towards the target. In other words, the two guide stars allow the telescope to track the target while the aircraft moves through the sky. To be selected as guide stars, they need to be sufficiently bright and not too close to the target so to prevent the imager from confusing the guide star with the target (provided that the target is also bright in the visible light). With time at a premium, Erik has just five minutes to get the telescope pointed at the target. Should any technical problem arise or if no guiding star is available in the field of view of the FPI+, the FFI camera can be used a tracking camera instead. Erik’s job is not limited to guiding the telescope. He has also to supervise the systems that keep the telescope stable and pointed despite of turbulence and vibrations induced by the airframe. Among them it is a set of fibre optics gyroscopes that are part of the control loop of the fine drive system, and contribute to keep the telescope pointed and inertially stable. One system he keeps an eye on is the cooling of the oil flow supplied to the hydrostatic bearing from a reservoir located in the forward cargo bay. By design, the gap between the bearing and housing has to be kept at 50 micron. A smaller gap would prevent the bearing from rotating the telescope; a wider gap would not allow a precise control and stabilization of the telescope and might produce leaks. This is why the temperature of the oil has to be within a certain range. This is the job of the cooling system whose regulation is based on the temperature of the cabin and use a counter-flow heat exchanger based on glycol. Should the need arise and only for minor issues, it is possible to carry out some simple inflight maintenance on the telescope hardware and even instrument. To do so, it requires stopping any observation, place the telescope in a safe configuration and avoid any manoeuvring. Providing that there is not turbulence, one of the two on board mechanics will approach the telescope and try to fix the issue.

Once the telescope has begun tracking and is stable, the science instrument team swing into action. As DSI engineer Dr. Christian Fischer explains, there are two types of specialists. One is responsible to oversee the overall health of the instrument while changing its settings throughout each leg to meet the requirements of the given observation. To illustrate, the instrument is like a camera and the instrument operator the photographer. By adjusting the camera’s settings, he can achieve shots with different effects. Any science instrument gathers such a vast quantity of raw data that it has to be reduced before being sent to the astronomer for analysis. However, during the flight, the astronomers can preview the raw data as it is being collected and determine if the observation is providing results of the expected quality. This is where the instrument scientist comes into play. Christian belongs to this category. Thanks to a keen interest in astronomy, he understands the astronomer’s language. Combined with a deep knowledge of how FIFI-LS works, he is able to help them get the most from the instrument. If the data is not satisfying the astronomers, he can advise on how to change the instrument’s settings to try achieving the wanted results. If instead the data is better than expected or has been gathered before the end of the leg, he and the astronomers can work together to determine a new set of settings for additional observations, exploiting to the fullest the time allocated on the leg.
The flawless execution of the flight plan and onboard activities are under the ever watchful eyes of the two mission directors. They oversee the progress of the observations and liaise with the pilots instructing them when to change course for a new observation leg or to compensate for large deviations from the nominal flight plan due to cross wind. Contrary to commercial airliners, SOFIA has to drift on the wind to maintain the telescope trained on the target for the whole duration of an observation leg. In other words, she has to fly a heading or angle rather than an established ground track. Every time SOFIA crosses a new airspace sector, the pilots ask the air traffic control (ATC) permission to freely move within 20 miles either side of the nominal ground track of the flight plan. As the pilots explains, the ATC is very cooperative in accommodating such a request and clear the airspace around SOFIA accordingly. In other words, SOFIA is free to manoeuvre within a 40-mile wide corridor without danger of colliding with another aircraft. Besides, at that altitude and time of the night there is little traffic. Typically, when the actual ground track has deviated from the nominal one by some 5 or 6 miles, the flight directors ask the pilots to turn the aircraft to return to the nominal ground track. Such changes in heading are small and within the range of movements of the telescope, so that the alignment with the target is not lost. Strong head or tail winds will shorten or stretch the length of a leg and this has to be accounted for during the flight itself to prevent traversing restricted airspaces or radical alteration of the remaining observation legs. As exposed during the mission briefing, we hit a few pockets of light turbulence. When we do so, I can appreciate how well designed the telescope stabilization’s system is. In fact, I can clearly perceive how it is aircraft that moves around the telescope, which remains stable and pointed at the target without feeling a hint of turbulence. In case of severe turbulence however, brakes would hold the telescope in place forcing it to move with the aircraft.
Ten hours is long flight, but they literally flight by. We started the observations at 11,000-meter altitude and, as we burned fuel and got lighter, we were able to raise our altitude to 13,000 meters. The higher the altitude, the better the quality of the infrared light detected. We land at dawn, just as the Sun is about to peek out the horizon. In this way, we have made the most of SOFIA’s endurance and maximized the observation time. Landing before dawn protects the aircraft in the case the door is jammed open. In fact, should sunlight enter the open cavity, the telescope would act as a magnifying glass producing a concentrated beam that might damage the structure of the aircraft. In a situation like this, landing before sunrise would give time to tow the aircraft into the hangar. If for any reason, the crew cannot return SOFIA at Palmdale, alternate airports are available throughout the whole flight. Should landing occur after sunrise and with the door open, the pilots would taxi to the hangar maintaining the telescope to the West, hence looking at the opposite portion of the sky from where the Sun is raising. We quickly disembark, everybody is tired and eager to catch some well-deserved sleep. As we leave the aircraft, Jerry and his crew are already swinging into action to prepare SOFIA for the next flight.
Maintaining a 40-year old aircraft no longer in production is not trivial. Sourcing as many spare parts is vital to guarantee that a structural or system problem will not jeopardize SOFIA’s operations. This is the responsibility of Michael Tobberman, manager of NASA Engineering Operations for SOFIA. Michael and his team are responsible for stockpiling spares, such as 6 working and no longer in production Pratt & Whitney JT9D engines. They also organise the logistics of sourcing components and make them available by the time the aircraft’s maintenance plan calls for their inspection. In this way, if a fault or damage is identified, a replacement would already be available, avoiding a flight cancellation or delay. Their work was made somewhat easier when they purchased a B747SP, now laying on a boneyard at the nearby Mojave airport. Should the need arise, they can quickly drive to Mojave and harvest the aircraft of the desire component. Back at Palmdale, they can fit it to SOFIA, in time for the evening mission. Michael also tells me how both Boeing and Pratt & Whitney are still providing valuable engineering and technical support related to the maintenance, respectively, of the airframe and engines. Furthermore, with some 10 B747SP still in service, Michael keeps in touch with their operators to exchange precious knowledge and in-service experience. Similarly to a commercial aircraft, SOFIA must undertake the so-called C-Checks, periods of heavy maintenance activities lasting several weeks. During a C-Check, the airframe is rigorously inspected and every defect assessed and repaired as appropriate. At the end of a C-Check, the airframe is basically good as new. Typically, a B747 undergoes a C-Check every 2 years. Because SOFIA flies only some 100 times a year, the FAA has granted her the so-called Low Usage Maintenance Program, or LUMP, which has extended the interval between C-Checks to some 6-7 years. This is a generous concession that greatly reduces the time the aircraft is grounded for maintenance and thus extends her observation time. While the last C-Check was performed by Lufthansa Technik in Frankfurt, Germany, the plan for the next one is to do it in Building 703, saving therefore the time and resources needed to ferry the aircraft to Europe.
The long-term well-being of the telescope assembly is DSI’s responsibility. Most of the companies that built its components are no longer in business or capable of offering customer support. Thus spare parts are hard to retrieve. As a remedy, the DSI has put together its own in-house maintenance capability. It consists of a workshop, set up and run by Marco Lentini, and an electronic lab managed by Alexander Steiner. The workshop is located outside of Building 703 and it allows Marco’s team to fabricate structural components and mechanisms. They cooperate closely with the electronic lab where they are specialized in reproducing almost every single electronic component belonging to the telescope, such as power units, computers, actuators, just to name a few. As Alexander explains, the health of the telescope is monitored on each flight. In this way, they can diagnose the behaviour of the individual components and determine when a replacement is needed. Thus, they can start build a new component, and have it ready by the time comes for its substitution. In fact, a better one. As technology has progressed since the telescope assembly was first built and with the advantage of years of operational experience, Alexander’s team is able of designing and building a component that is more reliable and capable that its predecessor. They are also animated by a proactive attitude, so that even if a component does not show signs of aging, they might kick off the design of an improved version ready to be fitted at the most convenient time. As the installation of any major component requires some flight testing before being cleared for operational usage, the electronic lab is equipped to carry out a vast array of simulations to test the component. The component is made to believe that it is mounted on the telescope and thus its behaviour can be monitored and any bug worked out before release for installation. In this way, flight testing is greatly reduced if not even completely dismissed. The lab operates in close conjunction with the hardware-in-the-loop lab. Here software upgrades are simulated and cleared before being uploaded into the telescope computers. Once again, such a process reduces flight testing to the minimum.
There is no doubt that operating SOFIA is a remarkable undertaking, an immiscible mix of engineering and science. What is the future of SOFIA? The observatory is slated to operate for 20 years. As she started operations in 2014, this means that we will see SOFIA flying at least up to 2034, funding permitting. As we have seen, operating a 40-year-old airframe carrying a 20-year-old telescope does have its challenges. It certainly begs the question if such resources are well spent. Do we need SOFIA when we have scores of telescopes in space? The answer is yes. Although an orbiting telescope can observe almost 24/7/365, once launched it can no longer be serviced and upgraded (the only exception was the Hubble Space Telescope when the Space Shuttle was still in service). SOFIA instead has this capability, as it returns home after every flight. This allows for the instruments to be upgraded and the aircraft to be periodically serviced. In other words, the whole observatory is continually being improved, modified and fixed. SOFIA will carry state-of-the-art instruments until when she is retired. One significant ramification is that more observations can be carried out within a given timeframe. An upgraded instrument is more efficient allowing for a reduction in observation time of a given target. Hence throughout a flight series, more targets can be studied. In a space-based telescope, where the instruments cannot be changed or upgraded, the observation time remains the same. In fact, it can lengthen as the instrument ages. Therefore, considering the same observation time, SOFIA is more productive if compared to a space-based telescope. Researchers can also take risks with technology that would be cost-prohibitive to trial on a space-based telescope. Hence SOFIA afford testing and refinement of instrument technology making it ready for installation onto a space-based telescope. While failures in space are not tolerated, with SOFIA you can work out the flaws and thus deliver a product that you know it will work in space. Lastly, SOFIA can quickly respond to astronomical events such as eclipses, supernova, and occultations, deploying where they will be visible. Space-based telescopes, constrained by orbital mechanics and limited consumables, rarely can offer such flexibility.
The evening after my flight I sit at the end of the runway waiting for SOFIA to depart on another science mission. As she begins the take-off run, the roar of the four engines fills the air but it quickly dissipates through the surrounding flat land. As she passes over me, the onboard lights lit her dimly so that I can admire her one last time. I bid her farewell for a long and productive life. Flying on SOFIA and observing first-hand what it takes to make her capable of accomplishing her mission has been one of the most rewarding experience I have ever had. I am grateful to all the people mentioned in this article who took time in explaining their work and answer my numerous questions. In particular, I wish to thank Dr. Dörte Mehlert for arranging the logistics of my visit and Dr. Markus Völter of the excellent Omega Tau science and technology podcast who put me in contact with the DSI.
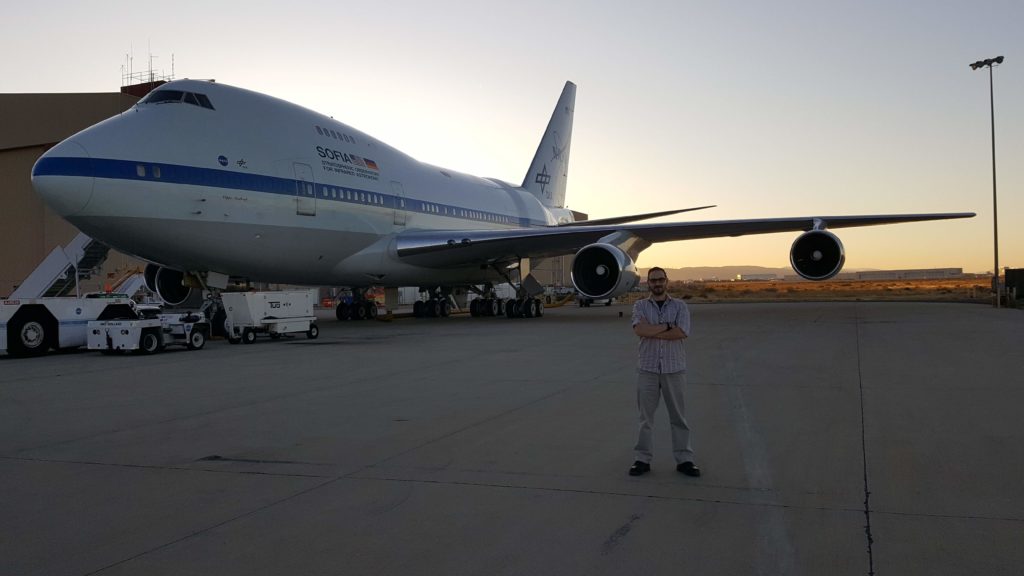
All rights reserved
Copyright: @ ADAA/Spazio Magazine/D.Sivolella